01.04.2025
3 minutes of reading
In the context of the energy transition, it is vital to study underground biogeochemical cycles given the pivotal role they play in H2 storage, geothermal energy, the extraction of critical metals and CO2 burial. Resident microbial communities have a significant influence on these environments, modifying permeability, for example, and rendering geothermal energy, gas storage and other such operations complex. Their metabolic activity can also cause damage, notably by corroding infrastructure and producing hydrogen sulfide.
Intense biogeochemical activity in the underground environment
Numerous research questions relating to the biogeochemical cycles governing the workings of the underground environment are now of fundamental importance in the context of the energy transition. From H2 storage to geothermal energy production, from the extraction of critical materials to CO2 burial, the underground carries with it some crucial challenges for our short- and medium-term future.
It is now acknowledged that subsurface environments can be greatly influenced by resident (prokaryotic) microbial communities [1]. In particular, the presence of bacteria and archaea1 organized in biofilms alters the permeability of the porous medium, hindering the operation of many applications, such as geothermal energy and geological gas storage [2]. Other studies indicate that 80% of injection wells in aquifers display clogging problems associated with bubble formation, suspended particles, microbial biofilm growth and induced mineral precipitation [3, 4]. In addition, the metabolic activity of bacteria and archaea present in the underground environment can consume and/or produce gases [5], thereby altering reservoir rocks [6] and, consequently, sometimes leading to major operational complications such as infrastructure corrosion, clogging of injection sites [7] and hydrogen sulfide production in gas storage facilities [8].
1 Single-celled microorganisms that are genetically distinct from bacteria
Impact of mineralogy and temperature on hydrogen consumption by subsoil microbial communities
For example, initial IFPEN research described in the source below focused on assessing the influence of mineralogy and temperature on hydrogen consumption by microbial communities typically found underground (methanogenic archaea, sulfate-reducing and homoacetogenic bacteria2). The research highlighted the importance of taking mineralogy and temperature into account when assessing potential underground hydrogen storage (UHS) reservoirs, as they can have a significant impact on indigenous microbial populations and their consumption dynamics with respect to this molecule. For example, the presence of gypsum favors sulfate reduction over methanogenesis and homoacetogenesis, especially at low temperatures, whereas methanogenesis is dominant at 34 and 40°C in the absence of sulfate (see Figure). At 25°C, homoacetogenic bacteria are favored over methanogenic archaea, and acetate production varies depending on the mineralogy of the rocks present. This suggests interactions between the microbial community and rocks, which can serve as substrates for biofilm formation. These metabolic changes are associated with radical shifts in microbial populations, highlighting the fact that ecosystem plasticity with respect to hydrogen also depends on the mineralogical composition of the reservoir.
2 Bacteria that use hydrogen and carbon dioxide to produce acetate
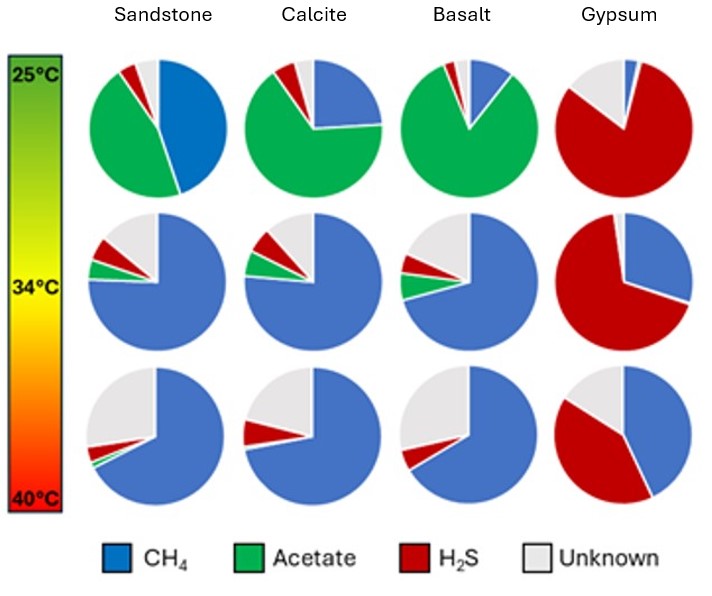
Experimental data for use in future modeling research
To further advance this research, IFPEN teams are carrying out a project aimed at developing multidisciplinary methodological approaches in order to gain a better understanding of the biogeochemical cycles at work underground and in the facilities operating there. New experimental set-ups are being developed, ranging from micromodels to coreflood3 via high-pressure reactors and calorimetry, to study biofilm dynamics and microbial and geochemical reactivity in porous media, at various scales and under conditions representative of the underground environment (temperature, pressure, flow). The data generated from this set of experiments will then be fed into modeling tools in order to improve the prediction of reactive phenomena in the underground environments studied.
Pour aller plus loin, les équipes d’IFPEN conduisent un projet visant à développer des approches méthodologiques multidisciplinaires pour mieux comprendre les cycles biogéochimiques ayant lieu dans les sous-sols et les installations qui y sont opérées. De nouveaux dispositifs expérimentaux sont développés, allant du micromodèle au coreflood3 en passant par les réacteurs haute pression et la calorimétrie, afin d’étudier la dynamique des biofilms et la réactivité microbienne et géochimique dans des milieux poreux, à différentes échelles et dans des conditions représentatives du sous-sol (température, pression, écoulement). Les données générées à partir de cet ensemble d’expérimentations alimenteront ensuite des outils de modélisation permettant d’améliorer la prédiction des phénomènes réactifs dans les environnements souterrains étudiés.
3 Experimental set-up for injecting a fluid or combination of fluids through a rock sample
Source:
Muller, E., Guélard, J., Sissmann, O., Tafit, A., & Poirier, S. (2024). Evidencing the influence of temperature and mineralogy on microbial competition for hydrogen consumption: Implications for underground hydrogen storage (UHS). International Journal of Hydrogen Energy, 82, 1101-1113.
>> https://doi.org/10.1016/j.ijhydene.2024.08.024
References:
[1] Rittenberg, S. C. (1964). Geological Microbiology: Introduction to Geological Microbiology. In Science, 143 (3611), 1156–1157.
>> https://doi.org/10.1126/science.143.3611.1156.b
[2] Gaol, C. L. et al. (2021). Investigation of clogging in porous media induced by microorganisms using a microfluidic application. Environmental Science: Water Research & Technology, 7(2), 441-454.
>> https://doi.org/10.1039/D0EW00766H
[3] Dillon et al. (1994). Review of International Experience in Injecting Water Into Aquifers for Storage and Reuse. Conference paper in Water Down Under 94: Groundwater Papers, Preprints of Papers, 13–19.
[4] Bloetscher, F. et al. (2014). Lessons Learned from Aquifer Storage and Recovery (ASR) Systems in the United States. Journal of Water Resource and Protection, 6 (17), 1603.
>> https://doi.org/10.4236/jwarp.2014.617146
[5] Dopffel, N. et al. (2021). Microbial side effects of underground hydrogen storage – Knowledge gaps, risks and opportunities for successful implementation. International Journal of Hydrogen Energy.
>> https://doi.org/10.1016/j.ijhydene.2020.12.058
[6] Trias, R. et al. (2017). High reactivity of deep biota under anthropogenic CO2 injection into basalt. Nature communications, 8(1), 1-14.
>> https://doi.org/10.1038/s41467-017-01288-8
[7] Klueglein, N. et al. (2016). Testing of H2S Inhibitors for Application in a MEOR Field Pilot in Germany. In SPE Improved Oil Recovery Conference. OnePetro.
>> https://doi.org/10.2118/179931-MS
[8] Hemme, C., & van Berk, W. (2017). Potential risk of H2S generation and release in salt cavern gas storage. Journal of Natural Gas Science and Engineering, 47, 114-123.
>> https://doi.org/10.1016/j.jngse.2017.09.007
Scientific contact : Elodie Muller
You may also be interested in
Colloid transport in porous media: deposits and plugging
The transport of colloidal particles in porous media is relevant to a number of fields, including geosciences and environmental engineering. Particle-matrix interactions can lead to deposit formation and accumulation, potentially damaging the medium and altering its permeability. (...) At IFPEN, the problem was originally studied for oil and gas production, but research has now been extended to include the fields of geothermal energy and geological storage of CO2.